What does superconductivity mean to semiconductors?
Recently, with the South Korean team's announcement of the LK-99 material, which is claimed to have the potential for room-temperature superconductivity, and its preparation method, a whirlwind has been stirred up in the global scientific community. Various teams are attempting to confirm (or refute) the room-temperature superconductivity characteristics of LK-99 from both experimental and theoretical perspectives.
The main reason why LK-99 has become the focus of attention in the global scientific community is that if room-temperature superconductivity is truly realized, it will greatly reduce the threshold for achieving superconductivity, thereby allowing a wide range of superconductivity-based applications to be widely used. Among these superconductivity-based applications, the one most closely related to the semiconductor industry is undoubtedly the quantum computer.
The main difference between quantum computers and conventional computers is that in conventional computers, each digital bit only represents 1 bit of information, which is either 0 or 1. All algorithms based on conventional computers (Turing machines) are designed based on this assumption, and in fact, this assumption has brought some limitations in computation, which has led to many important problems that cannot be solved within a reasonable time using Turing machine algorithms. These include a series of scientific computing problems (such as simulation of compound properties, simulation of quantum processes, etc.), optimization problems (such as the shortest path and optimal traffic planning, etc.), and decryption calculations. These problems are usually referred to as NP problems, which are problems that cannot be solved within polynomial time complexity using Turing machines (it is generally believed that problems with a polynomial relationship between computation time and scale can be completed within a controllable time, and if the computation time and scale are exponential, they cannot be completed within a reasonable time).
Advertisement
To solve these problems that conventional Turing machines cannot complete within a reasonable time, quantum computers have emerged. In quantum computers, instead of using unidirectional digital bits, quantum bits (qubits) are used. The biggest difference between quantum bits and traditional digital bits is that quantum bits can achieve state superposition, that is, a quantum bit can be in a 0 state or a 1 state at the same time, and only when the result is read out, it will return to 1 or 0 according to the probability distribution of each state. Therefore, by utilizing the magical properties of quantum bits, quantum computers can solve a series of NP problems within polynomial time, thus playing an extremely important role in important fields that require the use of NP algorithms (including the aforementioned scientific computing and optimization problems), allowing previously unsolvable problems to be solved precisely.
At present, top global research institutions such as Google, IBM, and IMEC have completed the preparation of quantum computer prototypes, and in these mainstream quantum computers, qubits are achieved through superconductivity. The specific principle is that qubits use a superconducting LC resonant network to achieve this. Such a superconducting LC network will have quantized energy states under the action of the Josephson effect, which can represent quantized 0s and 1s.
Before the advent of room-temperature superconductivity, superconductivity required very low temperatures (usually very close to absolute zero, such as the 10mK level). Under such conditions, quantum computers require huge cooling equipment, which limits the development and popularization of quantum computers. Therefore, if room-temperature superconductivity is truly realized, it will become an important driving force in this field.
Superconducting Quantum Computer Chip Design
As we all know, the main components of the current computer architecture (including processors, memory) are implemented by semiconductor chips, and in quantum computers that use superconductivity, semiconductor chips are also indispensable.As previously mentioned, qubits can be implemented using superconducting LC resonant circuits, and control of the qubits can be achieved by injecting different excitation signals into the superconducting LC circuits. Specifically, it is necessary to inject an AC signal (XY) and a DC signal (Z) into the LC resonant circuit of the qubit. The AC signal is typically a modulated pulse, while the DC signal requires precise control of the amplitude. Such qubit control is implemented through an ASIC chip based on semiconductor technology.
In the qubit control ASIC, the main function that the chip needs to achieve is high signal-to-noise ratio signal modulation: the XY path needs to generate pulses, while the Z path is mainly a DC signal. In fact, such requirements are already very common in current wireless communication applications, so the circuit design of the qubit control ASIC is actually very similar to that of RF circuits. For example, Google's quantum computing team released the design of its latest generation of qubit control ASIC circuits at this year's ISSCC. In terms of the XY path, the circuit architecture is very close to the IQ modulator transmitter in RF chips: first, the digital signal is converted into an analog baseband signal using a digital-to-analog converter (DAC) at the baseband, and then the analog baseband signal is upconverted to an RF frequency (usually in the range of 5-7 GHz) through an upconversion circuit, and such an RF modulated signal is used to control the XY path.
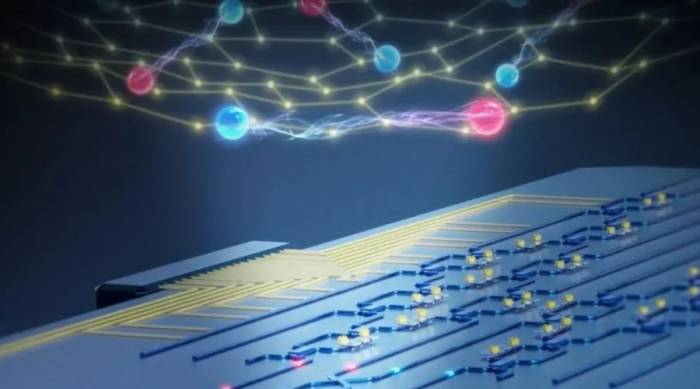
In terms of the Z path, since it is necessary to precisely control the DC value of Z, the circuit can use a digital-to-analog converter to directly convert the digital control signal into the corresponding analog signal.
It can be seen that quantum computing cannot do without semiconductor chips to control qubits, and such control is achieved by generating modulated pulse signals or DC signals, a process that is very close to wireless communication, so the mainstream qubit control circuits are also very similar to RF circuits in wireless communication.
Challenges of qubit control chip design
The design of qubit control chips can refer to existing RF circuits, but it also has its own challenges.Firstly, current superconductivity requires operation at temperatures that are almost at absolute zero, taking into account cooling equipment, the qubit control chip also needs to operate under conditions close to absolute zero (3-4 K). However, the low-temperature range of transistor modeling in the mainstream chip design PDK only covers down to minus 40 degrees Celsius (i.e., 233 K), which is far from the 3-4 K required for the qubit control chip. Without a good PDK model, it will inevitably bring challenges to the design of the qubit control chip. The first step for PDK is to solve the basic modeling problem, that is, under such low-temperature conditions, what is the behavior of a typical transistor (including current, noise, nonlinearity, etc.). After completing the basic modeling, PDK must also provide statistical modeling of transistor behavior under low-temperature conditions, including transistor mismatch, differences in transistor performance under different process corners. If we want to further expand the scale of quantum computers, it is necessary to use larger qubit control chips, then the modeling of process differences on large-scale chips will also become important. This step will be an important step for the qubit control chip to enter mass production.
At present, the design of superconducting quantum computers and related chips is mainly led by the relevant research departments of universities and technology companies (such as Google, IBM), and the commonly used semiconductor chip process is the mature 28nm. In order to truly enter mass production of superconducting quantum computers, relevant companies in the semiconductor industry (including EDA, foundries, etc.) must also be able to take corresponding actions. In this direction, Synopsis has formed a low-temperature chip R&D team with six other university research institutions in the UK, with Synopsis providing EDA capabilities based on TCAD to help complete the modeling of low-temperature semiconductors, with the goal of providing verified low-temperature semiconductor chip IP in the future, thereby accelerating the development of the entire superconducting quantum computer.
In addition to low temperature, the noise and nonlinear performance of the qubit control chip are also very important: a key indicator of a qubit is fidelity. In order to achieve an increase in the number of qubits, the fidelity of each qubit must reach 99.9% or higher, otherwise the quantum computer will not be able to perform effective calculations due to insufficient fidelity of each qubit; and the fidelity of the qubit is closely related to the noise and nonlinear performance of the qubit control chip. Since the qubit control chip has a similar architecture to the RF chip, the noise and nonlinear problems that occur in the RF chip will also appear in the qubit control chip. To ensure that the fidelity meets the standard, the signal-to-noise ratio (SDR) of the qubit control chip must reach more than 35dB, which requires each module in the qubit control chip (such as DAC, LO, etc.) to have good noise and linearity, and from an architectural perspective, it is also necessary to ensure that indicators such as LO leakage are sufficiently low.
Finally, considering cooling, the power consumption of the qubit control chip should not be too high. In low-temperature superconducting computers, if the power consumption of the qubit control chip is too high, the heat it emits will exceed the capacity of the cooling equipment, causing the temperature of the superconducting qubits to be too high to truly work in the superconducting state. Generally speaking, the power consumption of the qubit control chip needs to be controlled at 10-20 mW/qubit to meet the temperature control requirements.
If room-temperature superconductivity is achieved, it will promote the rapid development of qubit control chips.
The aforementioned superconducting quantum computers and qubit control chips need to operate in a temperature range close to absolute zero, and if room-temperature superconductivity (such as the superconductivity of LK-99 is truly verified) is achieved and can be used to manufacture qubits, superconducting quantum computers are expected to achieve leapfrog development. Under the condition of room-temperature superconductivity, the ultra-low temperature cooling equipment currently needed in quantum computers will no longer be used, which greatly reduces the threshold for preparing quantum computers and allows more institutions to have the opportunity to join the research and development of quantum computers.
From another perspective, even if the threshold for superconductivity is reduced, the demand for qubit control chips has not decreased: an important bottleneck of current quantum computers is the fidelity of qubits and related processing, so the performance of qubit control chips is crucial. If room-temperature superconductivity like LK-99 really becomes a reality and is used in quantum computers, we expect the following impact on quantum computer chips:
If the qubit control chip can work at room temperature, this reduces the requirements for modeling related chip devices (i.e., the design can be implemented using the PDK that has been verified at room temperature), and the power consumption requirements for the qubit control chip may also be more relaxed (since it only needs to work at room temperature, the demand for heat dissipation is reduced).
The demand for performance improvement of superconducting quantum computers will be greatly accelerated. From this perspective, the demand for qubit fidelity is higher (for example, if the number of qubits needs to be increased to thousands, 99.9% may not be enough, and it may need to reach 99.99% or even 99.999%), which puts higher demands on the performance of the qubit control chip: signal-to-noise ratio, linearity, etc., all need to be greatly improved to meet the demand.Finally, the circuit design of qubit control chips will further evolve to meet the needs of quantum computers. Qubit control chips will draw further inspiration from existing research on wireless communication chip circuits, while also needing to address their own unique challenges. With more and more research teams focusing on superconducting quantum computing, it is expected that this field will become a new hot direction in chip circuit research.
Leave A Comment